Author: Sunanda Biswas
Division of Soil Science and Agricultural Chemistry, ICAR-IARI, New Delhi-110012
*Corresponding author, email: sunandabiswas13@gmail.com
Introduction
In India, soil degradation (~ 57% of the cultivated area) is a major threat to agricultural sustainability and environmental quality both in irrigated and rain-fed agro-ecosystems. A perceived decline or stagnation in crop yield, partial factor productivity of inputs and also quality of the produce is the fall out of such degradation. Decline in soil organic matter and its associated nutrients supply in soil is the major factor for yield decline under intensive cropping systems. In addition, stresses due to acidity, salinity, alkalinity, and water logging etc. are also there for a considerable land area in different parts of the country. These degradative forces and processes impair soil’s essential ecosystem functions and ultimately its health/quality. Therefore, for sustainable use of soil and its protection against degradation soil quality assessment- fitness for use, and its resilienceâ€"ability to recover, and identification of diagnostic recovery modules are the only options available to address this critical issue. The concept of soil quality and the methods for evaluating it with respect to various soil functions have been evolved to a considerable extent for identifying major soil quality indicator. Though the indicators are put in use for monitoring soil quality (Andrews et al. 2004), such indicators for resilience purpose are yet to be developed to assess the ease and degree of recovery of degraded systems (Kuan et al. 2006). Theoretically, soil resilience has been defined as the capacity of soil to recover its functional and structural integrity after a disturbance (Lal 1997). Agriculture is one of the important stresses and disturbances to the soil environment (Brussard 1994). Although, there is a conceptual framework for evaluating soil resilience but there is limited field level validation. Soil resilience and resistance are affected by both inherent and dynamic soil characteristics and thus, will vary substantially from one area to the next and will change over time and management practices (Lal 1998). Management practices that increase soil organic matter levels will improve most soil functions. Thus, it is necessary to develop a protocol for evaluating soil resilience capacity of degraded soil with field level validation under different management interventions.
Principal processes involved in soil resilience
(a) Control of soil organic matter content
(b) Improvement in soil structure
(c) Increase in soil biodiversity
(d) Reduction in soil degradation and erosion rates below the soil formation rate
(e) Increase in nutrient capital and recycling mechanisms.
Factors affecting soil resilience
These factors are difficult to manage
• Soil Texture
• Soil Depth
• Soil Horizon Sequence
These factors can be managed
• Disturbance
• Diversity & Complexity
• Water, Nutrients & Energy
Soil functions involved in soil resilience
1. Nutrient cycling
2. Partitioning of water
3. Productivity
4. Water storage
5. Decomposition
6. Absorbing and detoxifying bacteria
7. Nutrient supplying capacity
Methods for estimation of soil resilience
Selection of sites and analysis of soils
GPS based soil sampling is to be done to select farmers’ field and soil samples are analysed for different physical (bulk density, clay content, mean weight diameter, aggregate stability water stable aggregate, saturated hydraulic conductivity), chemical (pH of soil, total soil organic carbon, easily oxidizable organic carbon, different fractions of OC, available nitrogen, available phosphorus, available potassium, available S, available Fe, Mn, Zn and Cu, available B, total nitrogen, cation exchange capacity and biological (microbial biomass C and N, mineralizable C and N, enzyme activity viz. dehydrogenase, acid and alkaline phosphatase, arylsulphatase, urease, Beta-glucosidase, FDA hydrolase activity) attributes of soil.
Screening of soil quality indicators
To select a representative minimum data set (MDS) (Andrews et al., 2002) only those soil properties that shows significant treatment differences are selected. The principal components receiving high eigen values and variables with high factor loading are assumed to be variables that best represented system attributes. Therefore, only the PCs with eigen values > 1 (Brejda et al., 2000) and those that explained at least 5% of the variation in the data are examined. Within each PC, only highly weighted factors are retained for MDS. Highly weighted factor loadings are defined as having absolute values within 10% of the highest factor loading. When more than one factor is retained under a single PC, multivariate correlation coefficients are employed to determine if the variables can be considered redundant and therefore eliminates from the MDS (Andrews et al., 2002). Well-correlated variables are considered redundant and only one is considered for the MDS. The rest are eliminated from the data set. If the highly weighted variables are not correlated, each is considered important and is retained in the MDS. As a check of how well the MDS represents the management system goals, multiple regression is performed using the indicators retained in the MDS as independent variables and the end point measures like percent relative yield of Kharif crop, Rabi crop and mean relative yield of the cropping systems as dependent variables. If any variable within the MDS do not contribute to the coefficient of determinant of multiple regressions of the variables, it is also dropped from the MDS and the remaining indicators are considered as master indicators.
Soil quality index
After determining the MDS indicators, every observation of each MDS indicator is transformed using a linear scoring method (Andrews et al., 2002). Indicators are arranged in order depending on whether a higher value was considered ‘‘good’’ or ‘‘bad’’ in terms of soil function. For ‘more is better’ indicators, each observation is divided by the highest observed value such that the highest observed value received a score of 1. For ‘less is better’ indicators, the lowest observed value (in the numerator) is divided by each observation (in the denominator) such that the lowest observed value received a score of 1. Each PC explained a certain amount (%) of the variation in the total data set. This percentage, divided by the total percentage of variation explained by all PCs with eigenvectors >1, provides the weighted factor for variables chosen under a given PC. Then sum up the weighted MDS variables scores for each observation using the following equation:
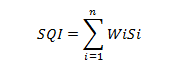
Where, S is the score for the subscripted variable and Wi is the weighing factor derived from the PCA. Here the assumption is that higher index scores means better soil quality or greater performance of soil function.
Soil resilience Index
Based on this quantitative value of the key indicators, SQI values are calculated for all the experimental sites. On the basis of soil quality assessment data management interventions are selected. The resilience index (RI) of the soils of each of the selected sites after receiving management intervention is calculated using the following expression
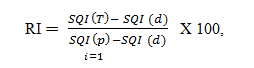
Where, SQI (T): The SQI value of soil after management intervention (T)
SQI (d): The SQI value of soil before the management intervention (I)
SQI (p): The computed SQI value of pristine soil near the corresponding site.
The numerator in the above expression indicates the recovery of SQI value due to management intervention where as the denominator indicates the loss of SQI value due to soil degradation processes.

Schematic model for soil resilience measurement
Potential indicators of soil resilience
1. Soil structure
2. Microaggregates
3. Retention and transmission properties
4. CEC
5. Exchangable cations
6. Soil organic matter content
7. Transformations
8. Nutrient supplying capacity
9. Soil pH
10. Rooting depth
11. Soil biodiversity
12. Soil fauna activity
13. Microbial activity
Conclusion
Soil quality is assessed integrating physical, chemical and biological attributes of soils. These attributes might be changed under the influence of various management practices and cropping systems and as such aggrade or degrade soil quality. The conceptual framework for evaluating soil resilience has been designed with field level validation. The method described here for measuring soil resilience can successfully be used to measure the resilience power of degraded soils in response to management interventions.
References:
1. Andrews, S.S., Karlen, D.L., Cambardella, C.A., 2004. The soil management assessment framework: a quantitative soil quality evaluation method. Soil Sci. Soc. Am. J. 68, 1945â€"1962.
2. Andrews, S.S., Karlen, D.L., Mitchell, J.P., 2002. A comparision of soil quality indexing methods for vegetable systems in Northern California. Agric. Ecosyst. Environ.90, 25â€"45.
3. Brejeda, J. J, Moorman, T. B., Karlen, D.L., Dao, T. H., 2000. Identification of regional soil quality factors and indicators.I.Central and southern high plains. Soil Sci. Soc. Am. J. 64, 2115-2124.
4. Brussard, L. 1994. Interrelationships between biological activities, soil properties and soil management. In Soil Resilience and Sustainable Land Use, eds. D.J. Greenland and I. Szabolcs, 309-352, CAB Int. Wallingford, Oxon, UK.
5. Kuan, H.L., P.D. Hallett, B.S. Griffiths, A.S. Gregory, C.W. Watts, and A.P. Whitmore.
2006. The biological and physical stability and resilience of a selection of Scottish soils to stresses. European Journal of Soil Science 58(3): 811-821.
6. Lal, R. 1997. Degradation and resilience of soils. Philosophical Transactions of the Royal
Society of London B 352: 997-1008.
7. Lal, R. 1998. Soil quality and sustainability. In Methods for Assessment of Soil Degradation,eds. W.H. Blum, C. Valentine and B.A. Stewart, 17-30, CRC Press, Boca raton, FL.
About Author / Additional Info:
WORKING AS A SCIENTIST IN ICAR-IARI